Evaluation de l'effet des paramètres affectant l'élimination des phosphates biologiques et physico-chimiques des eaux usées dans un système multi-couches de sol
Résumé
L’évacuation des eaux usées est un problème qui s’aggrave dans certaines zones rurales marocaines. Des rejets de phosphore et d’azote élevés peuvent entraîner l’eutrophisation des eaux réceptrices. Le processus biologique est l’alternative la plus adaptée aux besoins de ces zones, comme le système des filtres imbriqués (FI). Le procédé d’épuration des eaux usées en milieu rural par FI, un système innovant utilisé pour la première fois au Maroc, a été étudié en modélisant les relations entre un ensemble de facteurs environnementaux et le phosphore total éliminé, sur la base de 153 échantillonnages. Trois modèles pilotes de FI, construites dans trois boîtes en plastique de 36 cm x 30 cm × 65 cm , ont été alimentés en continu par des eaux usées domestiques, avec un taux de charge hydraulique (HLR) de 250, 500 et 1000 l / m2/ jour. Cette étude a pour but d’étudier et de quantifier l’effet des paramètres affectant l’élimination biologique et physico-chimique du phosphore des eaux usées dans ce système, à l’aide de réseaux neuronaux (NN) et d’analyses de régression multiple (ARM). Les résultats montrent l’influence de la charge hydraulique (HLR), du potentiel hydrogène (pH), de la charge de phosphore (PL), du nitrite (NO2--N), de l’oxygène dissous (OD), de la demande biochimique d’oxygène (DBO5) et du nitrate (NO3--N) dans l’élimination du phosphore avec une contribution de 36, 16, 15, 12, 9, 7 et 6 % respectivement.
Mots clés: Analyse de régression multiple, filtres imbriqués, les réseaux de neurones, Phosphore.
Téléchargements
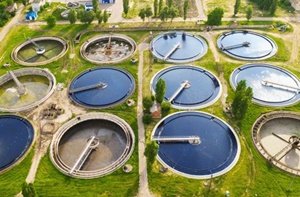
Publié-e
Numéro
Rubrique
Licence
Revue Marocaine des Sciences Agronomiques et Vétérinaires est mis à disposition selon les termes de la licence Creative Commons Attribution - Pas d’Utilisation Commerciale - Partage dans les Mêmes Conditions 4.0 International.
Fondé(e) sur une œuvre à www.agrimaroc.org.
Les autorisations au-delà du champ de cette licence peuvent être obtenues à www.agrimaroc.org.