Production d'acide lactique à partir de peau et de chair de banane non mûrie par saccharification et fermentation simultanées
Résumé
Le but de cette étude est d’établir un processus de production d’acide lactique (LA) à partir de deux différents types de déchets organiques en Afrique, à savoir la pelure de bananes non-matures en utilisant comme souche modèle Lactobacillus pb pentosus AH 239. La bioconversion du glucose contenu dans la biomasse d’acide lactique (LA) a été réalisée après le processus de saccharification et fermentation simultanées (SSF). L’Hydrolyse séparé et la fermentation (SHF) ont été également appliqués dans cette étude pour comparer l’efficacité des deux processus. Les résultats ont montré que le rendement de l’hydrolyse enzymatique a été significativement amélioré en cas d’un SSF enregistrant un taux d’hydrolyse dans l’intervalle de 82%-90% contre 52%-61% dans les conditions SHF. Les résultats montrent également que la SSF donne une production plus efficace d’acide lactique avec un rendement supérieur à 90%, et une concentration élevée jusqu’à 50 g/L. En raison de sa performance, le processus de SSF pour la production d’acide lactique peut être un moyen important de bioconversion de résidus lignocellulosiques en Afrique. L’optimisation de ce processus doit être adaptée au contexte africain et pour son développement à l’échelle industrielle.
Mots clés: Déchets organiques, Processus SSF, Processus SHF, Acide lactique, Lactobacillus bp Pentosus AH 239, Pelure de banane.
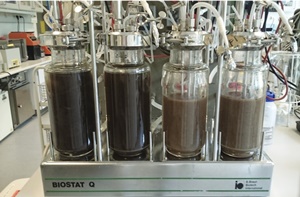
Publié-e
Comment citer
Numéro
Rubrique
Licence
© Revue Marocaine des Sciences Agronomiques et Vétérinaires