Variabilité macromorphologique de la morelle jaune (Solanum elaeagnifolium Cav.) et sa relation avec le climat et l’altitude au Maroc
Résumé
La relation entre certains traits morphologiques et le potentiel invasif des plantes exotiques envahissantes a été signalée. En plus, l’invasion biologique est souvent associée à une évolution rapide des espèces introduites. L’objectif de cette étude a été d’établir la structure de la variabilité morphologique de Solanum elaeagnifolium et de vérifier si l’invasion au Maroc a été suivie par une évolution rapide qui pourrait affecter son potentiel invasif. Au total, 709 individus issus de 218 sites présentant différentes conditions écologiques ont été caractérisés pour 12 variables. L’Analyse Ascendante Hiérarchique et l’Analyse Discriminante Linéaire, ont pu établir quatre morphotypes. Les traits liés au nombre total de branches et de baies sont ceux qui discriminent le plus entre les morphotypes. La description des quatre morphotypes implique une évolution rapide de cette espèce au Maroc. Cette évolution a donné naissance à de grands plants avec un nombre de baies très élevé. Cependant, les plants de taille réduite prédominent et sont les plus invasifs. La répartition géographique des quatre morphotypes indique que S. elaeagnifolium est une plante r-stratégiste, particulièrement dans les conditions écologiques les plus sévères. Cette stratégie lui permet d’allouer la grande partie de son énergie à la reproduction et de devenir plus invasive par conséquent.
Mots-clés: Solanum elaeagnifolium, traits morphologiques, potentiel invasif, morphotypes, facteurs écologiques.
Téléchargements
Full-text of the article is available for this locale: English.
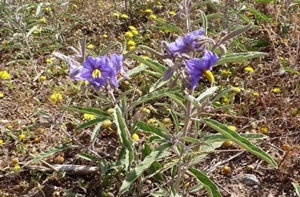
Téléchargements
Publié-e
Comment citer
Numéro
Rubrique
Licence
Revue Marocaine des Sciences Agronomiques et Vétérinaires est mis à disposition selon les termes de la licence Creative Commons Attribution - Pas d’Utilisation Commerciale - Partage dans les Mêmes Conditions 4.0 International.
Fondé(e) sur une œuvre à www.agrimaroc.org.
Les autorisations au-delà du champ de cette licence peuvent être obtenues à www.agrimaroc.org.